
disscusion about a story
Experiment 4Characterization of α-Lactalbumin, a Milk Proteinα-Lactalbumin is one of the major proteins found in milk. Its function is to regulate the synthesisof the milk sugar lactose. It can be isolated readily from milk and purified by Sephadex gelfiltration or affinity chromatography. This experiment introduces the student to a series of proteinpurification steps that yield α-Lactalbumin in sufficient amounts and purity for further physicalcharacterization by UV spectroscopy and polyacrylamide gel electrophoresis.Introduction and TheoryProtein purification is an activity that has occupied the time of biochemists throughout the historyof biochemistry. In fact, a large percentage of the biochemical literature is a description of howspecific proteins have been separated from the thousands of other proteins and biomolecules intissues, cells, and biological fluids. Biochemical investigations of all biological processes require,at some time, the isolation, purification, and characterization of a protein. Of course, there is nosingle technique or sequence of techniques that can be followed to purify all proteins. Mostinvestigators approach the problem by trial and error. Fortunately, the experiences and discoveriesof hundreds of biochemists have been combined so that today, a general and somewhat systematicapproach is used for protein purification. The best purification procedure is one that yields amaximum amount of the purified protein in a minimum amount of time. The following discussionoutlines the basic steps that must be considered in order to develop a protein purification scheme(Table 1).Development of Protein AssayFirst and foremost in any protein purification scheme is the development of an assay for theprotein. This procedure, which may have a physical, chemical, or biological basis, is necessary inorder to determine quantitatively and/or qualitatively the presence of the specific protein. Duringthe early stages of purification, the particular protein desired must be distinguished from thousandsof other proteins present in crude cell homogenates. The desired protein may be less than 0.1% ofthe total protein content of the crude extract. If the desired protein is an enzyme, the obvious assaywill be based on biological function, that is, a measurement of the enzymatic activity after eachisolation-purification step. If the protein to be isolated is not an enzyme or if the biological activityof the protein is unknown, physical or chemical methods must be used. One of the most usefulanalytical methods is electrophoresis.Source of the ProteinThe selection of a source from which the desired protein is to be isolated should be considered. Ifthe objective is simply to obtain a certain quantity of a protein for further study, you would choosea source that contains large amounts of the protein. The best choice is an organ from a large animalthat can be obtained from a local slaughterhouse. Microorganisms are also good sources becausethey can be harvested in large quantities. However, if you desire a specific protein from a specifictype of cell, tissue, cell organelle, or biological fluid, the source is limited. If the desired protein isknown to be located in an organelle or sub compartment of the cell, partial purification of theprotein is achieved by isolating the organelle. If the protein is, instead, in the soluble cytoplasmof the cell, it will remain dissolved in the final supernatant obtained after centrifugation at 100,000x g.With the introduction of new technology in DNA recombinant research, it is possible to transfer,via a plasmid, the specific gene for a protein into another organism (usually Escherichia coli cells)and allow that organism to synthesize the desired protein.Table 1: Typical Sequence for the Purification of a Protein
Develop an assay for the desired protein.
Select the biological source of the protein.
Release the protein from the source and solubilize it in an aqueous buffer system.
Fractionate the cell components by physical methods (centrifugation).
Fractionate the cell components by differential solubility.
Ion-exchange chromatography.
Adsorption chromatography.
Gel filtration.
Affinity chromatography.
Isoelectric focusing.Preparation of a Crude ExtractOnce the protein source has been selected, the next step is to release the desired protein from itsnatural cellular environment and solubilize it in aqueous solution. This calls for disruption of thecell membrane without damage to the cell contents. Proteins are relatively fragile molecules andonly gentle procedures are allowed at this stage. The gentlest methods for cell breakage are osmoticlysis, gentle grinding in hand-operated glass homogenizers, and disruption by ultrasonic waves.These methods are useful for “soft tissue” as found in green plants and animals. When dealing withbacterial cells, where rigid cell walls are present, the most effective methods are grinding in amortar with an inert abrasive such as sand or alumina, treatment with lysozyme, or both. Lysozymeis an enzyme that catalyzes the hydrolysis of polysaccharide moieties present in cell walls. Whenvery resistant cell walls are encountered (yeast, for example), the French press must be used. Herethe cells are disrupted by passage, under high pressure, through a small hole. Osmotic lysisconsists of suspending cells in a solution of relatively high ionic strength. This causes water insidethe cell to diffuse out through the membrane. The cells are then isolated by centrifugation andtransferred to pure water. Water rapidly diffuses into the cell, bursting the membrane. Anotheralternative, gentle grinding, is best accomplished with a glass or Teflon homogenizer. Thisconsists of a glass tube with a close-fitting piston. The cells are forced against the glass walls underthe pressure of the piston, and the cell components are released into an aqueous solution. Ultrasonicwaves, produced by a sonicator, are transmitted into a suspension of cells by a metal probe. Thevibration set up by the ultrasonic waves disrupts the cell membrane, releasing the cell componentsinto the surrounding aqueous solution.A less gentle, but widely used, solubilization device is the common electric blender. This methodmay be used for plant or animal tissue, but it is not effective for disruption of bacterial cell walls.Since so many cell disruption methods are available, the experimenter must, by trial and error, finda convenient method that yields the maximum quantity of the protein with minimal damage to themolecules.Stabilization of Proteins in a Crude ExtractContinued stabilization of the protein must always be considered in protein purification. While theprotein is inside the cell, it is in a highly regulated environment. Cell components in thesesurroundings are protected against sudden changes in pH, temperature, or ionic strength andagainst oxidation and enzymatic degradation. Once the cell wall barrier is destroyed, the protectiveprocesses are no longer functional and degradation of the desired protein is likely to begin. Anartificial environment that mimics the natural one must be maintained so that the protein retainsits chemical integrity and biological function throughout the purification procedure. What factorsare important in maintaining an environment in which proteins are stable? Although numerousfactors must be considered, the most critical are (1) ionic strength and polarity, (2) pH, (3) thepresence or absence of metal ions, (4) oxidation, (5) endogenous proteases, and (6) temperature.The standard cellular environment is, of course, aqueous; because of the presence of inorganicsalts, though, its ionic strength is relatively high. Addition of KC1, NaCI, or MgCl2 to the cellextract may be necessary to maintain this condition. Proteins that are normally found in thehydrophobic regions of cells (i.e., membranes) are generally more stable in aqueous environmentsin which the polarity has been reduced by addition of 1 to 10% glycerol or sucrose.The pH of a biological cell is controlled by the presence of natural buffers. Since protein structureis often irreversibly altered by extremes in pH, a buffer system must be maintained for proteinstabilization. The importance of proper selection of a buffer system cannot be overemphasized.Obviously, a “perfect” buffer system is not available, but some are much better (more natural) thanothers. Even at this advanced stage in our understanding of solution buffering, trial and error arestill essential in selecting an effective, noninterfering buffer system. For most cell homogenates atphysiological pH values, tris and phosphatebuffers are widely used.The presence of metal ions in mixtures of biomolecules can be both beneficial and harmful. Metalions such as Na+, K+, Ca2+, Mg2+, and Fe3+ may actually increase the stability of dissolved proteins.Heavy metal ions such as Ag+, Cu2+, Pb2+, and Hg2+ are deleterious, particularly to proteins thatdepend on sulfhydryl groups for structural and functional integrity. The main sources ofcontaminating metals are buffer salts, water used to make buffer solutions, and metal containersand equipment. To avoid heavy metal contamination, you should use high-purity buffers, glassdistilled water, and glassware specifically cleaned to remove extraneous metal ions. If metalcontamination still persists, a chelating agent such as ethylenediaminetetraacetic acid (EDTA, 1 x10-4 M) may be added to the buffer. This, of course, should not be used if the desired protein is anenzyme that is inhibited by EDTA.Many proteins are susceptible to oxidation. This is especially a problem with proteins having freesulfhydryl groups, which are easily oxidized and converted to disulfide bonds. A reducingenvironment can be maintained by adding mercaptoethanol, cysteine, or dithiothreitol (1 x 10-3 M)to the buffer system.Many biological cells contain degradative enzymes (proteases) that catalyze the hydrolysis ofpeptide linkages. In the intact cell, functional proteins are protected from these destructiveenzymes because the enzymes are stored in cell organelles (lysosomes, etc.) and released onlywhen needed. The proteases are freed upon cell disruption and immediately begin to catalyze thedegradation of protein material. This detrimental action can be slowed by the addition of specificprotease inhibitors such as phenylmethylsulfonyl fluoride or certain bioactive peptides. Theseinhibitors are to be used with extreme caution because they are potentially toxic.Many of the above conditions that affect the stability of proteins in solution are dependent onchemical reactions. In particular, metal ions, oxidative processes, and proteases bring aboutchemical changes in proteins. It is a well-accepted tenet in chemistry that lower temperatures slowdown chemical processes. We generally assume that proteins are more stable at low temperatures.Although there are a few exceptions to this, it is fairly common practice to carry out all proceduresof protein isolation under reduced-temperature conditions (0 to 4°C).After cell disruption, gross fractionation of the properly stabilized, crude cell homogenate may beachieved by physical methods, specifically centrifugation.Separation of Proteins Based on Solubility DifferencesProteins are soluble in aqueous solutions primarily because their charged and polar amino acidresidues are solvated by water. Any agent that disrupts these protein-water interactions decreasesprotein solubility because the protein-protein interactions become more important. Protein-proteinaggregates are no longer sufficiently solvated, and they precipitate from solution. Because eachspecific type of protein has a unique amino acid composition and sequence, the degree andimportance of water salvation vary from protein to protein. Therefore, different proteins precipitateat different concentrations of precipitating agent. The agents most often used for proteinprecipitation are (1) inorganic salts, (2) organic solvents, (3) polyethylene glycol (PEG), (4) pH,and (5) temperature. The most commonly used inorganic salt, ammonium sulfate, is highlysolvated in water and actually reduces the water available for interaction with protein. Asammonium sulfate is added to a protein solution, a concentration of salt is reached at which thereis no longer sufficient water present to maintain a particular type of protein in solution. The proteinprecipitates or is “salted out” of solution. The concentration of ammonium sulfate at which thedesired protein precipitates from solution cannot be calculated but must be established by trial anderror. In practice, ammonium sulfate precipitation is carried out in stepwise intervals. For example,a crude cell extract is treated by slow addition of dry, solid, high-purity ammonium sulfate in orderto achieve a change in salt concentration from 0 to 25% in ammonium sulfate, is gently stirred forup to 60 minutes, and is subjected to centrifugation at 20,000 x g. The precipitate that separatesupon centrifugation and the supernatant are analyzed for the desired protein. If the protein is stillpredominantly present in the supernatant, the salt concentration is increased from 25 to 35%. Thisprocess of ammonium sulfate addition and centrifugation is continued until the desired protein issalted out. Although other inorganic salts are used, ammonium sulfate has many advantages,including high solubility in water and less chance of causing protein denaturation.Organic solvents also decrease protein solubility, but they are not as widely used as ammoniumsulfate. They are thought to function as precipitating agents in two ways: (1) by dehydratingproteins, much as ammonium sulfate does, and (2) by decreasing the dielectric constant of thesolution. The organic solvents used (which, of course, must be miscible with water) includemethanol, ethanol, and acetone. Organic solvents must be used with care because they are morelikely than ammonium sulfate to cause protein denaturation. A relatively new method of selectiveprotein precipitation involves the use of nonionic polymers. The most widely used agent in thiscategory is polyethylene glycol. The polymer is available in a variety of molecular weights rangingfrom 400 to 7500. The biochemical literature reports successful use of different sizes, but lowermolecular-weight polymer has been shown to be the most specific. The principles behind the actionof PEG as a protein precipitating agent are not completely understood. Possible modes of actioninclude (1) complex formation between protein and polymer and (2) exclusion of the protein frompart of the solvent (dehydration) followed by protein aggregation and precipitation. This methodof protein precipitation should receive considerable attention in the future because it has severaladvantages over other fractionation techniques. Possibly the most attractive advantage of PEG isits ability to fractionate proteins on the basis of size and shape as in gel nitration. Although it istoo early to state a general rule, most fractionation experiments with PEG show that the larger theprotein, the less soluble it is in highly concentrated polymer solution.Finally, changes in pH and temperature have been used effectively to promote selective proteinprecipitation. A change in the pH of the solution alters the ionic state of a protein and may evenbring some proteins to a state of charge neutrality. Charged protein molecules tend to repel eachother and remain in solution; however, neutral protein molecules do not repel each other, so theytend to aggregate and precipitate from solution. A protein is least soluble in aqueous solution whenit has no net charge, that is, when it is isoelectric. This characteristic can be used in proteinpurification, since different proteins usually have different isoelectric pH values.An increase in temperature generally causes an increase in the solubility of solutes. This generalrule is followed by most proteins up to about 40°C. Above this temperature, however, manyproteins aggregate and precipitate from solution. If the protein of interest is heat stable and stillwater soluble above 40°C, a major step in protein purification can be achieved because most otherproteins precipitate below 40°C and can be removed by centrifugation.With so many different protein-fractionating methods available, it becomes difficult to choose thecorrect one. As a guideline, it is fair to state that all precipitation methods discussed will providesome purification for most proteins. Their effectiveness is nearly the same, so the selection shouldbe based on the convenience of the method and the stability of the desired protein under theexperimental conditions.Selective Techniques in Protein PurificationAfter gross fractionation of proteins, as discussed above, more refined methods with greaterresolution can be attempted. These methods, in order of increasing resolution, are ion-exchangechromatography, adsorption chromatography, gel filtration, affinity chromatography, andisoelectric focusing. Since the basis of protein separation is different for each of these techniques,it is often most effective and appropriate to use all of the techniques in the order given.Chromatographic methods for protein purification are discussed in your text. However, we mustconsider another important topic, preparation of protein solutions for chromatography.Fractionation of heterogeneous protein mixtures by inorganic salts, organic solvents, or PEGusually precedes ion-exchange chromatography. The presence of the precipitating agents willinterfere with the later chromatographic steps. In particular, the presence of ammonium sulfateincreases the ionic strength of the protein solution and damps the ionic protein-ion exchange resininteractions. Procedures that are in current use to remove undesirable small molecules from proteinsolutions include ultrafiltration, dialysis, and gel filtration (size exclusion). All three methodseffectively remove small molecules without serious damage to or loss of proteins.Chromatography is now and will continue to be the most effective method for selective proteinpurification. The more conventional methods (ion exchange and gel filtration) rely on rathernonspecific physico-chemical interactions between a stationary support and protein molecule.These techniques, which separate proteins on the basis of net charge, size, and polarity, do nothave a high degree of specificity. All of these methods must be attempted during the purificationof a new protein.The highest level of selectivity in protein purification is offered by affinity chromatography—theseparation of proteins on the basis of specific biological interactions. A modified form of affinitychromatography, immobilized metal-ion affinity chromatography (IMAC), was introduced byPorath in 1975. In this method, the bioactive support consists of metal ions chelated to an insolublematrix. A typical affinity support for IMAC, iminodiacetic acid (IDA) covalently linked toagarose, is shown in Figure 1. Proteins are separated according to their individual abilities to bindto the immobilized metal ions. Electron-donating amino acid side chains on the surfaces of proteinmolecules interact with the metal ions. Important electron-rich groups on the protein include theimidazole ring of histidine, the indole ring of tryptophan, and the sulfhydryl group of cysteine.These amino acid side chains are able to displace weakly bound ligands such as water. A typicalimmobilized metal ion as shown in Figure 1 may have up to three adsorption sites for proteinbinding. Proteins bound to affinity supports may be displaced by free ligands, such as imidazole,ammonia, pyrophosphate, or amino acids, that bind to the metal ion.Adsorption sitesFigure 1: Structure of metal ion-IDA-agarose affinity support. Me = metal ion such as Cu(ll),Zn(ll), Ni(ll), or Fe(lll).To some individuals, especially those who recall their experiences in organic chemistry laboratory,the ultimate step in purification of a molecule is crystallization. The desire to obtain crystallineprotein has long been powerful and many proteins have been crystallized. However, there is acommon misconception that the ability to form crystals of a protein ensures that the protein ishomogeneous. For many reasons (entrapment of contaminants within crystals, aggregation ofprotein molecules, etc.), the ability to crystallize a protein should not be used as a criterion ofpurity. However, protein crystallization is a worthwhileendeavor in the final stages of protein purification because it would be expected to remove minorimpurities. The interest in protein crystals today has its origin in the demand for X-raycrystallographic analysis of protein structure.Purification of α-LactalbuminMilk is composed of several proteins, including the caseins (phosphoproteins), β-lactoglobulin, αlactalbumin, albumin, and immunoglobulins. A crude preparation of α-lactalbumin was firstobtained from milk in 1899. Since that time, several investigators have developed procedures forisolating and purifying the protein. α-Lactalbumin has a molecular weight of 14,178 Da andcontains 129 amino acid residues. It is present in milk at concentrations averaging 1 mg/mL andwas at first thought to serve a nutritional function. We now know that it is an essential componentof the lactose synthase system. Thorough discussion of the biological role of α-lactalbumin isavailable in the literature (Ebner, 1970).The primary proteins in milk are the caseins. These may be removed from milk by acid and heatinduced precipitation at pH 4.6 followed by centrifugation. The remaining supernatant (calledwhey) contains primarily α-lactalbumin and β-lactoglobulin. These two proteins may be separatedby gel filtration (size exclusion chromatography) or with a Cu(II)-IDA-agarose affinity column.The specific amino acid side chains on α-lactalbumin responsible for binding to the metal supportare not known; however, α-lactalbumin is a metalloprotein. Under physiological conditions, itcarries one Cu(II) per molecule; hence, there are metal binding sites on the protein. Column-boundα-lactalbumin is eluted by a solution of the free ligand imidazole. A flowchart outlining theseprocedures is shown in Figure 2.Nonfat Milk → centrifuge → discard sediment, keep supernatant → change pH to 4.6,heat to 40 C and centrifuge → discard sediment, keep supernatant (whey) → sephadexchromatography → α-lactalbumin Characterization by SDS polyacrylamide electrophoresisFigure 2: Flowchart for isolation and purification of α-lactalbumin.Analysis and Characterization of α-LactalbuminRecall from the previous discussion of protein purification that an important step is thedevelopment of a specific assay for the desired protein. The assay must be specific so that thedesired protein can be detected both quantitatively and qualitatively in a solution containingthousands of other proteins. This is difficult for α-lactalbumin because it has no unique physical,chemical or biological property that can be readily measured. Measuring its biological activity asa modifier protein in the lactose synthase system is the most specific way to analyze α-lactalbumin;however, the assay, which requires a coupled enzyme system and preparation of numeroussolutions, is too time-consuming to complete in this laboratory period.Ebner, K. E. (1970). “Biological role for alpha-lactalbumin as a component of an enzyme requiring two proteins”.Acc. Chem. Res. 1970; 3(2): 41-47Two useful methods for the quantitative determination of protein solutions were discussed inExperiment 1. The Bradford protein assay will be used to determine the total protein concentrationin the α-lactalbumin preparations once chromatography has been completed.Additionally, most proteins have a broad characteristic absorption spectrum centered at around280 nm. The major absorption is due to the presence of aromatic moieties in the amino acidsphenylalanine, tyrosine, and tryptophan. During the α-lactalbumin purification described in thisexperiment, you will monitor the process by measuring the absorption at 280 nm (A280) of columnfractions to be sure the experiment is proceeding correctly. All proteins absorb light at 280 nm dueto the fact that the conjugated pi electrons of aromatic amino acids are excited at this wavelength.The protein-containing fractions from column chromatography will be identified by measurementof the absorption at 280 nm. This will not identify which protein is present, it will only tell uswhich fractions contain protein(s). Thus, the chromatography procedure outlined here will providea final product that has an ultraviolet absorption at 280 nm, indicating that protein material is likelypresent in solution. However several questions remain unanswered:
How much protein was isolated?
What is the purity of the protein sample?
What is the identity of the protein?Neither Bradford assay nor absorption at A280 are specific for a certain type of protein; rather theyboth estimate total protein content. The Bradford can help us answer question 1 above. However,it is unlikely that the protein fractions from this experiment contain a single type of protein. Howmany different proteins are present? What is the relative abundance of each protein? Is αlactalbumin the predominant protein in the isolated fractions? These questions may be answeredby analysis of the isolated fractions by electrophoretic methods. The technique of polyacrylamidegel electrophoresis (described below) will be introduced and applied to the column-purifiedfractions, crude whey fraction, and standard α-lactalbumin, in order to separate the proteinscontained in each sample based on molecular weight. Determining the molecular weights of eachprotein found in the samples is useful for identifying which protein it is (by comparing to literaturevalues). However, it is usually important to do further experiments to reliably determine theidentity of an isolated protein. A common method employed to specifically identify a protein isWestern blotting. This method is further described below, and we will employ this method toconfidently determine if we have managed to isolate/purify α-lactalbumin.Polyacrylamide Gel ElectrophoresisProteins can be separated on the basis of their size in a similar way to how DNA fragments can beseparated through agarose gel electrophoresis, which you may be familiar with from moleculargenetics classes. Electrophoretic separation of biomolecules is a central and fundamental processin the life sciences such as biochemistry and molecular genetics, and many other fields.In electrophoresis, mixtures of molecules (DNA, RNA or Protein) can be separated by loadingthem into the wells of a porous gel, and applying an electrical field to the gel. The gel consists ofa meshwork of pores, and is submerged in an electrophoresis buffer which is able to conductelectrical current.Molecules will migrate through the gel towards the cathode or anode, depending on their charge.I.e. negatively charged molecules migrate towards the positive anode. The distance and rate atwhich the molecules travel is a function of their molecular weight, charge (positive or negative)and their shape. Larger molecules will have a harder time traveling through the pores of the gel,so they do not travel as far or fast as smaller molecules, which have an easier time getting throughthe mesh of pores. If all the different molecules have the same shape (for example linear) and samecharge, then only the molecular weight affects migration (Figure 3).Figure 3: Electrophoresis scheme. Samples are loaded into the sample wells at the top of the gel. Electrical currentis applied across the gel and negatively charged molecules migrate towards the positive electrode. The rate of theirmigration is a function of their molecular weight, smaller molecules travel easier through the pores and end upfurther down the gel. Larger molecules struggle more to pass through the pores, causing a slower rate of migrationand do not migrate as far as smaller molecules. If the molecules are not in their linear form, then their shape alsoaffect migration rate.Electrophoresis of nucleic acids does not require much pretreatment of the sample, other thanmixing it with loading dye (aka “loading buffer”), because DNA and RNA are negatively chargedin their natural state. Simply loading the sample with loading dye into the gel and applying currentwill cause these molecules to migrate towards the positive electrode. The distance they travel is apure function of their length/molecular weight, since they will in most circumstance all have thesame shape (linear). The loading dye consists mainly of glycerol and a dye such as bromophenolblue. The glycerol is there to weigh the sample down (it is much denser than water), allowing it tofall into the appropriate well in the gel, and not float out again before the current is applied. Theblue dye is used to allow the technician to see that the sample is going into the gel – it makesloading the gel much easier, and also allows us to track the progress of the electrophoresis.However, when electrophoresing proteins, one must consider the fact that proteins in their naturalstate have both positively and negatively charged residues, and also that proteins are usually foldedin various 3D shapes. Therefore, simply placing proteins in a gel and applying current, would notallow those proteins to separate solely based on their size, and their migration direction would alldepend on each specific proteins shape, size and charge.To overcome the problems of varying charge and shape, protein samples are treated with SodiumDodecyl Sulfate (SDS), which is a synthetic organic compound with formula CH3(CH2)11SO4Na.SDS is an anionic detergent that binds quantitatively to proteins, denatures/linearizes them, andimposes a large negative charge that overwhelms any charges the proteins may have. Thus all theproteins in a mixture now have an equal negative charge-to-mass ratio and can be separated in thesame way as described above for nucleic acids. SDS binds to proteins through hydrophobicinteraction of the saturated hydrocarbon chain of SDS (refer to your text book for the chemicalstructure of SDS) and the protein’s hydrophobic regions. The SDS is contained in the loading dyewhich is mixed with the sample before loading the gel. The loading dye also contains glycerol anda dye (often bromophenol blue) for same reasons as described above for agarose gelelectrophoresis.Once the sample and the loading dye have been combined in appropriate amounts, the solution isheated at 94°C for a few minutes, in order to allow the proteins to denature and react with the SDS.Although SDS is a denaturing agent in itself, some proteins are resistant to SDS and require heatingto initiate denaturation. For similar reason, the loading dye also contains reducing agents such asDithiothreitol (DTT) or 2-β-mercaptoethanol to facilitate denaturation. 2-β-mercaptoethanolprevents oxidation of cysteines and breaks up disulfide bonds. Once the proteins have been treatedwith loading buffer, they are loaded into the wells of a polyacrylamide gel similar to that seen inFigure 3, and electrophoresed.The technique for electrophoresis of proteins is referred to as Sodium Dodecyl Sulfate PolyAcrylamide Gel Electrophoresis, or simply SDS-PAGE. For most proteins, agarose is not useful,because the pores are too large (approx. 150nm) to obtain good resolution. Therefore, polymerizedacrylamide is used. Acrylamide in its polymerized form provides a meshwork of pores withappropriate size (3-6nm) for separating proteins, and is also sometimes used for resolving verysmall nucleic acid fragments. Figure 4 shows what polymerized acrylamide looks like under anelectron microscope.Figure 4: Porous mesh structure of a polyacrylamide gel as seen under scanning electron microscopy. Imageadopted from Patras et al. 2000.SDS-PAGE gels consist of several components, and usually the gel is divided into two parts/layers:The top layer has wells that the samples are loaded into and consists of a lower percentage ofpolyacrylamide. Less polyacrylamide produces a more porous gel, i.e. a gel that has less restrictionon protein migration. This layer is referred to as the “stacking gel” and acts to concentrate largesample volumes, resulting in better band resolution than is possible using the same volumes on agel without a stack. Once the migrating proteins reach the bottom of the stacking gel, they enterthe other part of the gel, referred to as the “resolving gel”. The resolving gel consists of the samecomponents as the stacking gel, but has a higher percentage of polyacrylamide, which causes theproteins to separate with high resolution on the basis of their sizes. Gels with stacking andresolving parts are referred to as a “discontinuous polyacrylamide gels”. Without thisdiscontinuous system, all the proteins would not enter the gel as a narrow band. Rather they wouldmove into the gel a different times, resulting in poor resolution and smeared bands.To make a polyacrylamide gel; acrylamide, bis acrylamide, Tris-HCl buffer and SDS are firstmixed together, then TEMED and ammonium persulfate (APS) are added to the solution. Thesetwo last reagents form a free radical-generating system which causes the polymerization ofacrylamide and bis-acrylamide (Figure 6), i.e. they become cross-linked, producing the porousmesh structure of polyacrylamide seen in Figure 4. A higher amount of acrylamide/bis-acrylamideproduces a gel with more cross linking, and hence a more dense structure that is harder to migratethrough.Figure 6: The copolymerization of acrylamide and bis-acrylamide catalyzed by Ammonium persulfate andTEMED. Figure adopted from https://www.nationaldiagnostics.com/electrophoresis/article/polyacrylamide-matrix,accessed 08/01/2017.Traditionally, after electrophoresis is finished, the gel is submerged in a solution of Coomassieblue. This dye attaches to the proteins making it possible to see how the molecules have migrated.A more modern method uses “stain-free” gels. These gels contain trihalo compounds which reactwith tryptophan and produce fluorescence when activated by UV-light, which can be detected witha Gel Doc or ChemiDoc imager. This modern technology is available to us.Western blottingThe term “blotting” refers to the transfer of biological samples (DNA, RNA or protein) from anelectrophoresis gel onto a membrane and the subsequent detection of the molecules on themembrane using probes that specifically bind to a molecule of interest.Blotting was first reported in 1975 by British biologist Edwin Southern, whom employed themethod to analyze DNA samples. The method is referred to as “Southern blotting”, eponymouslynamed after its inventor. Subsequently, RNA blotting was developed by James Alwine et al. in1977 and the name “Northern blotting” was coined as a play on the Southern blotting name. InSouthern and Northern blotting, labeled oligonucleotide probes are used to detect specific DNAsequences and RNA transcripts that have been blotted onto the membrane.Finally, blotting of proteins was introduced by Tobin et al. in 1979, and termed “Western blotting”,which again was a play on the Southern blotting name. In Western blotting, proteins are firstseparated by size using SDS-page, then electrophoretically transferred to a membrane(nitrocellulose or Polyvinylidene fluoride, PVDF) and then the blotted proteins are detected usingantibodies specific towards the target protein. Such antibodies can be produces synthetically, ormore commonly they are produced by inoculating an animal with the protein of interest whichelicits an immune-response in the animal and production of protein-specific antibodies. Theseantibodies are then harvested and purified (usually by affinity chromatography) and conjugated toa molecule that can be detected. Commonly, the conjugated molecule is an enzyme, such as horseradish peroxidase (HRP) that will colorimetrically develop a color visible to the naked eye whenexposed to a substrate. Other molecules used for the same purpose include radioactive tags,fluorescent tags and alkaline phosphatase.Western blotting is now a routine technique for protein analysis in many fields of the life-sciencessuch as biochemistry, cancer biology, immunogenetics, recombinant protein expression,immunology and others. The method is also used in medical diagnostics, for example as aconfirmatory HIV test to detect HIV-antibodies in a patient’s blood. Thus, western blotting is notonly useful for detecting proteins, but also for detecting antibodies. Western blotting is also usedin definitive testing for variant Creutzfelt-Jacobs disease, which is a type of prion diseasecontracted by consumption of meat from cows with Bovine Spongiform Encephalopathy (madcow’s disease).Although an SDS-page gel stained with Coomassie in itself does provide useful evidence for thepresence of a target protein in a sample, and can even provide comparative data on the expressionlevel of a protein (say for example comparing expression of protein X in cancer cells versus normalcells), the information obtained is based on the known molecular weight of the target protein. Aprotein band of expected molecular weight in the gel implies that the target protein is there.However, as many proteins can be of similar molecular weight, the mere presence of a band at theexpected molecular weight in a gel does not actually prove that the target protein is present. Thisis why western blotting is so useful; the antibody will only bind to the target, which providesdefinitive evidence that the target protein is present.The major steps of Western blotting explained:
Electrophoresis: Protein containing solution is electrophoresed in an SDS-page gel to separate theproteins based on molecular weight.
Transfer/blotting: The separated proteins are transferred (blotted) onto a nitrocellulose or PVDFmembrane by sandwiching the gel and membrane together and performing an electrophoresis inthe direction perpendicular to the SDS-page electrophoresis direction (Figure 1). This makes theproteins leave the gel and blot onto the membrane.
Blocking: Nitrocellulose and PVDF membranes have a very strong affinity for protein. The blottedproteins are at this point stuck to the membrane nearly irreversibly. The next major step is toincubate the membrane with the antibody, so that the antibody can bind to the target protein.However, consider the following: Antibodies are also protein, and the membrane with happily bindany protein. Thus, without appropriate treatment of the blotted membrane, the antibodies can bindto anywhere on the membrane itself, thus becoming useless for detecting the target protein.Therefore, before incubating the membrane with antibody, the membrane must be “blocked”, suchthat the antibodies only have the option of binding to protein and not membrane. Blocking isachieved by incubating the blotted membrane in a buffer solution (Tris buffered saline) thatcontains non-target proteins. These non-target proteins will bind all over the parts of the membranethat do not have blotted proteins, thus inhibiting the antibodies from binding randomly and nonspecifically to the membrane.Most commonly, skim milk powder (which contains a large range of different proteins) is mixedwith the buffer to make up the blocking solution. This works great for most applications, but nowconsider this problem relating to our experiment: we are trying to detect a specific milk protein,and that protein will most certainly be found in skim milk powder. Hence, for our application, skimmilk powder will not work; it will probably just result in the antibody binding to many places onthe membrane, making it impossible to say with certainty that we have detected alpha-lactalbumin!Luckily there is an alternative to skim milk powder for blocking the membrane: Bovine SerumAlbumine (BSA). The blocking buffer we will use in our experiment contains BSA instead of skimmilk powder.
Antibody incubation: After blocking the membrane to avoid non-specific binding of the antibody,the membrane is incubated in a buffer with the exact same formulation as the blocking buffer, butwith added antibody. In the majority of western blotting experiments, two different antibodies areused in two separate and subsequent incubations. The first antibody (“primary antibody”) is the onethat is specific to the target protein. Once this incubation is completed, non-bound/unreactedantibodies are washed away with buffer, and then the second antibody (“secondary antibody”)incubation takes place. The secondary antibody is an antibody that has affinity for the primaryantibody (not the target protein) and has the conjugated HRP or other detection molecule. Thismethod is referred to as the indirect method, and is by far the most common way to do westernblotting. Alternatively, the primary antibody itself can be conjugated with the detection molecule,and no secondary antibody is necessary. This method is referred to as the “direct method”, andsaves time, resources and money. So why then bother with the double antibody sandwich?? Oneadvantage of the indirect method is that more than one secondary antibody can bind to the primaryantibody, resulting in an amplification of the detection signal, which can be vital for success in anexperiment with low concentration of protein (Figure 2).
Wash step(s): After each antibody incubation, it is necessary to remove any antibody moleculesthat have not reacted with the target protein. This is done by washing the membrane in anappropriate buffer (TBS for example) that contains a low percentage of detergent such as Tween20. Failure to wash may result in a lot of background signal developing after the HRP substrate isadded, because non-reacted antibody molecules are likely to be lying around on the membrane,although they have not reacted with any protein.
Signal development: The final step is to remove the wash buffer, and then cover the membranewith the substrate for the conjugated enzyme. In our experiment, the antibody is conjugated to HRP,which catalyzes the conversion of a chromogenic substrate (TMB, DAB or ABTS) into a coloredproduct in the presence of an oxidizing agent such as hydrogen peroxide. Alternatively, HRPproduces light when reacting with chemiluminescent substrates such as Luminol. The latter methodis referred to as Enhanced Chemiluminescence by Luminol (ECL).Figure 1: A) SDS-page to separate proteins in sample, B) Transfer of the separated proteins from the gelto the membrane, C) Detection of the target protein with antibody. Image adopted from: https://www.biorad-antibodies.com/western-blotting-immunoblotting-introduction.htmlFigure 2: Western blotting direct method vs. indirect method. Image adopted from:https://www.thermofisher.com/us/en/home/life-science/protein-biology/protein-biology-learningcenter/protein-biology-resource-library/pierce-protein-methods/overview-western-blotting.htmlOverview of the ExperimentThe complete isolation, purification, and characterization of α-lactalbumin as described hererequire about 9 hours. The preparation of whey is completed in approximately 3 hours and thechromatographic step (Sephadex or affinity) requires another 3 hours. The analysis proceduresrequire 3 hours. The whey fraction may be stored in a freezer for several weeks if desired.In week one of this experiment, we will prepare milk whey and prepare chromatography columnsto be used to following week. In week 2 the α-lactalbumin will be purified from whey usingchromatography. In the third week, we will analyze the fractions obtained in week one and twousing Bradford assay and SDS-PAGE. In the fourth week we will gain final evidence that alphalactalbumin was in fact isolate/purified, by employing Western blotting.Experimental ProcedureWeek 1: Preparation of Milk Whey & Preparation of Sephadex ColumnA. Preparation of Milk WheyObtain 10 mL of nonfat milk and centrifuge at 16,000 x g for 50 minutes in a refrigeratedcentrifuge. (During this step, proceed directly to the chromatography step.) Decant the supernatantinto a small beaker. The sediment and floating lipid layer should remain in the centrifuge tube andbe discarded. Adjust the pH of the supernatant to 4.6 in two steps:
Adjust to approximately pH 5 with dropwise addition of 12 M HC1.
Adjust to the desired pH (4.6) with dropwise addition of 0.5 M HC1.Heat the coagulated solution with constant stirring at 40°C for 30 minutes. Centrifuge at 16,000 xg for 30 minutes. Collect the whey (supernatant) and clarify by filtering through a 0.45 µm syringecartridge filter. The whey is stored for short periods of time (hours) in crushed ice or for longerperiods in a freezer.Make sure to also keep a sample of whole milk (1ml) and crude milk (100ul). Crude milk is whatis obtained after the first centrifugation. We will use these in our analysis in week 3 of theexperiment.B. Sephadex ChromatographyPacking the Sephadex ColumnSephadex G-50 has been prepared for your use. The dry beads have been pre-swollen in water forseveral hours, fine particles that may slow column flow have been removed, and the gel has beenequilibrated in 0.02 M Tris buffer, pH 7.0. Using the glass columns provided, close the stopcockand add 10 to 15 ml of Tris buffer into the column. Pour a well-mixed slurry of Sephadex G-50into the column and allow the column to pack with a very slow flow rate. As the gel settles into abed, add more slurried G-50 until the settled bed reaches a height of at least 30 cm. During thispacking process, never allow the column to run dry. If more buffer solution is necessary, add itcarefully without disturbing the gel. After a bed of desired height has been prepared, continueeluting the column with buffer. Add the flow adapter to the top of the column as instructed. Becareful not to disturb the gel at the top of the column. (End week 1 of experiment)Week 2: Sephadex ChromatographySample ApplicationMeasure out 3-4 mL of whey for application to the column. After the eluting solvent has justdrained into the top of the G-50 column, add the α-lactalbumin solution dropwise to the top of thegel. Use a pipet and avoid disturbing the top surface of the gel. After the protein solution has justentered the gel, slowly and carefully add 1.0 mL of buffer to the top of the column. Do not disturbthe gel surface. Allow the sample to penetrate the surface of the column. Repeat once more withanother 1.0 ml. Then, immediately add buffer to fill the column. Set up an eluting solvent supplythrough the flow adapter and adjust the hydrostatic pressure to give you a flow rate of 1 drop everyten seconds. Collect 1 mL samples and transfer the contents of every second 1 mL sample to aUV-cuvette and measure the absorbance of 280 nm wavelength light. For a blank, use 0.02 M Trisbuffer. Record the A280 values vs. mL of eluant in your notebook. When you begin to detectprotein eluting from the column (A280 > 0.10), measure the A280 of every fraction, and make a plotof A280 vs. mL of eluant. Continue collecting fractions until no more protein is eluted (about 30fractions). Save peak fractions that have A280 over 0.2. When you are finished with the Sephadexcolumn, pour the gel into a container labeled “used Sephadex G-50.” The gel may be recycled andused later.Analysis of ResultsStudy your experimentally derived plot of A280 vs. ml of elutant. How many major protein peaksare present? Which peak has the greatest amount of protein? Do you believe that each peakcontains a single type of protein, or does it contain a mixture? If each peak represents a mixtureof proteins, what one thing does each protein have in common? The presence of α-lactalbuminin a fraction cannot be confirmed until further characterization. However, if you know that αlactalbumin is among the smallest proteins in milk (molecular weight is 14,178Da), can youdetermine which protein peak contains α-lactalbumin? You may want to seach internet to findout the molecular weight of β-lactoglobumine in order to answer this question (End week 2 ofexperiment).Week 3: SDS-PAGESDS-PAGE Gel, Buffers and Reagents1) SDS-PAGE GelWe will use commercially available ready-made gels from BioRad (catalogue number 4568094).Make sure to visit biorad.com note the product details so you can include them in your report.2) Reagents for loading buffer. Store at room temp (5 mL of each)a) 10% SDS. Dissolve 0.5 g SDS in water and bring to 5 ml with dH2Ob) Glycerolc) 2-ß-mercaptoethanold) 0.1% (w/v) bromophenol blueSee below for exact composition of the loading buffer3) 5X Electrode (Running) Buffer, pH 8.3Tris base 4.5 gGlycine 21.6 gSDS 1.5 gMake to 300 ml with distilled water. Store at 4°C.Combine 60 mL of 5x electrode buffer with 240 mL of water to get 1x concentration for use inelectrophoresis (what is the final concentration (g/L) of Tris, Glycine, and SDS in the 1xelectrode buffer? Be sure to include this in your Materials and Methods section).ProcedurePreparation of sample for SDS-PAGE1) Make loading buffer (sample buffer) by adding the following reagents together:Distilled water 2.0 ml0.5 M Tris-HCl, pH 6.8 0.5 mlGlycerol 0.4 ml10% (w/v) SDS 0.8 ml2-ß-mercaptoethanol 0.2 ml0.1% (w/v) bromophenol blue 0.1 ml4.0 mlCAUTION: Open and keep 2-ß-mercaptoethanol in the fume hood! It is very volatile and causesheadaches if the fumes are inhaled. The prepared loading buffer should be stored and used in thehood.2) The loading/sample buffer is 2x concentrated and should be mixed with the sample at a 1:1ratio. 25µl of the sample+buffer should be loaded into the gel.3)Remove the gel assembly from the packaging and carefully remove the green comb to exposethe wells. Remove the green tape from the bottom of gel. Failure to do this will inhibit the electricalcurrent and nothing will move through the gel. Your instructor will demonstrate this step.4) Rinse each well completely with distilled water. Take care not to damage the wells. The gelsare now ready to be attached to the inner cooling core, the samples loaded, and the gels run.5) The protein samples should be diluted at least 1:1 with sample buffer and then heated at 95°Cfor 4 minutes. Total volume (sample with sample buffer) to load in each lane is 25ul. It is wise toprepare slightly more than what you will need, for example 30ul. The molecular weight standardis ready to use and does not require any preparation.6) Assemble upper buffer chamber. Make sure the U shaped inner cooling core gaskets areclean. It is very important that there is good contact at the point where the short glass platecontacts the notch in the gasket to prevent upper buffer leaks. Two groups must now share asingle inner cooling core.Loading sample wells1) Obtain 300 ml of electrode buffer by combining 60 mL of 5x electrode buffer with 240 mL ofwater. This amount will be sufficient for both groups sharing the gel apparatus. Alternativelyyour instructor may already have done this – make sure to check!2) Lower the inner cooling core into the lower buffer chamber of the Mini-PROTEAN II cell.Add approximately 11 mL buffer to the upper buffer chamber. Fill until the buffer reaches alevel halfway between the short and long plates. Do not overfill the upper buffer chamber.3) Pour the remainder of the buffer into the lower buffer chamber so that at least the bottom 1 cmof the gel is covered. Remove any air bubbles from the bottom of the gel using the bent pipet sothat a good electrical contact is achieved (the instructor will demonstrate).4) Load the prepared samples into the wells under the electrode buffer using the gel loading tipsfor the pipette. Insert the tip to about 1-2 mm from the well bottom before delivery. Make suresample does not flow out of one well and into another. Protein standards should be placed onleft-most or right-most side of the gel.Running the gel1) Place the lid on top of the lower buffer chamber such that colors match. Attach electricalleads to power supply and begin electrophoresis. Run gels at 200 volts, constant voltage setting,for approximately 45 min. The exact time required to run the gel can vary (see step 2 below).2) When dye front reaches near the end of the gel (do not let the dye front run off thebottom of the gel), turn off power supply and disassemble apparatus. The gel should bemonitored carefully during the electrophoresis, or else the dye will run off the bottom making ithard to determine the relative mobility values of proteins (see subsequent section).3) Carefully unsnap one face of the cassette, leaving the gel adhering to the other face. Use thespatula to carefully release the gel from the sides of the cassette, then transfer your gel to the gelimaging camera and capture an image of the gel.Analysis of Electrophoresis Results:Study the gel and estimate the number of protein components in each lane. Take a picture of thegel (alternatively draw a diagram of your gel). For your report, the gel should be properly labeledand captioned. Measure the distance traveled by each protein (including the molecular weightstandard proteins and all other protein species). Using the values for molecular weight standardbands, plot a graph of the log of molecular weight (y-axis) vs. distance travelled (x-axis).Obtain the equation for the linear trend line. Use the equation for the linear trendline todetermine the molecular weight of each protein species in the sample lanes. Note that you doneed to calculate the MW of each and every band in each individual lane, since each lane willcontain the same proteins, although some lanes should have fewer types of proteins than others.Make sure that you have attempted to identify each protein species seen in the gel.In your results, include the graph, and a table of the distances and molecular weights for sampleproteins and molecular weight standards. Try to determine the identity of each protein in yourgel. You will have to consult literature for this, and you should cite/reference any sources ofinformation you use. Citing/referencing web pages is acceptable, if peer review literature cannotbe found.82% of the proteins in milk are caseins. There should be major differences between the originalmilk and our whey preparation. Of the remaining 18% of milk proteins that are found in theprepared whey, β-lactoglobulin is the most abundant followed by α-lactalbumin and then bloodalbumins.The molecular weight standards are random proteins that may or may not be found in milk (seebelow, obviously egg ovalbumin would not be found in cow’s milk). The molecular weightstandards could be for example: aprotinin from bovine lung (6,500), α-lactalbumin from bovinemilk (14,200), trypsin inhibitor from soybean (20,000), trypsinogen from bovine pancreas(24,000), carbonic anydrase from bovine erythrocytes (29,000), glyceraldehyde-3-phosphatedehydrogenase from rabbit muscle (36,000), egg ovalbumin (45,000), and bovine albumin(66,000). The standards may change from year to year. This year the molecular weigh standard“Precision Plus Protein Standards – Dual Color” from BioRad (catalogue # 161-0374) was used.Make sure to visit BioRad.com to figure out exactly which proteins it contains and whattheir molecular weights are. Your gel should be properly and clearly presented with themolecular weight of each band in the molecular weight ladder, well/lane numbers, figurenumber, figure title and a caption explaining which sample is in which well.Further Analysis (include in the results section):Use the α-lactalbumin and β-lactoglobulin standards in your SDS-page gel to:a. Estimate the total protein concentration in the prepared sampleb. Estimate the yield (mass amount) of purified α-lactalbumin in the prepared samplec. Estimate the concentration of α-lactalbumin in your purified sampled. Estimate of the % purity of α-lactalbumin preparation. Use the standards of α-lactalbuminand β-lactoglobulin to estimate the concentration of the different proteins in the sampleand divide the estimated α-lactalbumin concentration by the concentration of all proteinscombined, and then multiply by 100 to get % puritye.Week 4: Western blottingWhile watching the video, note the procedural steps, composition and concentration of reagentsused, and include this info in your report. Western blotting results should be presented in thesame way as SDS-page results.Points to consider when writing your report:Your Discussion section should discuss the following:1) What evidence do you have to claim that α-lactalbumin was (or was not) obtained?2) How much (µg) α-lactalbumin was obtained in the fractions selected from thechromatography?3) What is the concentration of α-lactalbumin in your selected fraction(s) fromchromatography?4) How successful was the purification of α-lactalbumin? Contemplate the purity of theprepared α-lactalbumin. If you have not managed to obtain entirely pure α-lactalbumin,what is/are the likely reason(s) for this? Which specific part(s) of the purificationprocedure may have been done poorly causing this? Explain what could have been donebetter to obtain higher purity. Your biochemistry text-book may contain usefulinformation about this. Keep in mind that there are four parts to the purification scheme:centrifugation, acid treatment, heat treatment and chromatography. A meaningfuldiscussion requires consideration of the identity of any contaminating proteins.Your Introduction section should explain all the concepts pertaining to purification of αlactalbumin, how SDS-page works to separate proteins and how Western blotting works to detecta specific protein. A good lab report will explain the function of all the reagents used in SDSPAGE and western blotting.Procedure and Rational section should as always be a complete account of the workperformed, such that another scientist could repeat your work exactly how you did it. Be sure toprovide info on the exact products/reagents/etc you used.
Get professional assignment help cheaply
Are you busy and do not have time to handle your assignment? Are you scared that your paper will not make the grade? Do you have responsibilities that may hinder you from turning in your assignment on time? Are you tired and can barely handle your assignment? Are your grades inconsistent?
Whichever your reason may is, it is valid! You can get professional academic help from our service at affordable rates. We have a team of professional academic writers who can handle all your assignments.
Our essay writers are graduates with diplomas, bachelor, masters, Ph.D., and doctorate degrees in various subjects. The minimum requirement to be an essay writer with our essay writing service is to have a college diploma. When assigning your order, we match the paper subject with the area of specialization of the writer.
Why choose our academic writing service?
Plagiarism free papers
Timely delivery
Any deadline
Skilled, Experienced Native English Writers
Subject-relevant academic writer
Adherence to paper instructions
Ability to tackle bulk assignments
Reasonable prices
24/7 Customer Support
Get superb grades consistently
Get Professional Assignment Help Cheaply
Are you busy and do not have time to handle your assignment? Are you scared that your paper will not make the grade? Do you have responsibilities that may hinder you from turning in your assignment on time? Are you tired and can barely handle your assignment? Are your grades inconsistent?
Whichever your reason may is, it is valid! You can get professional academic help from our service at affordable rates. We have a team of professional academic writers who can handle all your assignments.
Our essay writers are graduates with diplomas, bachelor’s, masters, Ph.D., and doctorate degrees in various subjects. The minimum requirement to be an essay writer with our essay writing service is to have a college diploma. When assigning your order, we match the paper subject with the area of specialization of the writer.
Why Choose Our Academic Writing Service?
Plagiarism free papers
Timely delivery
Any deadline
Skilled, Experienced Native English Writers
Subject-relevant academic writer
Adherence to paper instructions
Ability to tackle bulk assignments
Reasonable prices
24/7 Customer Support
Get superb grades consistently
How It Works
1. Place an order
You fill all the paper instructions in the order form. Make sure you include all the helpful materials so that our academic writers can deliver the perfect paper. It will also help to eliminate unnecessary revisions.
2. Pay for the order
Proceed to pay for the paper so that it can be assigned to one of our expert academic writers. The paper subject is matched with the writer’s area of specialization.
3. Track the progress
You communicate with the writer and know about the progress of the paper. The client can ask the writer for drafts of the paper. The client can upload extra material and include additional instructions from the lecturer. Receive a paper.
4. Download the paper
The paper is sent to your email and uploaded to your personal account. You also get a plagiarism report attached to your paper.
PLACE THIS ORDER OR A SIMILAR ORDER WITH Essay fount TODAY AND GET AN AMAZING DISCOUNT
The post Biochemical investigations of all biological processes appeared first on Essay fount.
What Students Are Saying About Us
.......... Customer ID: 12*** | Rating: ⭐⭐⭐⭐⭐"Honestly, I was afraid to send my paper to you, but you proved you are a trustworthy service. My essay was done in less than a day, and I received a brilliant piece. I didn’t even believe it was my essay at first 🙂 Great job, thank you!"
.......... Customer ID: 11***| Rating: ⭐⭐⭐⭐⭐
"This company is the best there is. They saved me so many times, I cannot even keep count. Now I recommend it to all my friends, and none of them have complained about it. The writers here are excellent."
"Order a custom Paper on Similar Assignment at essayfount.com! No Plagiarism! Enjoy 20% Discount!"
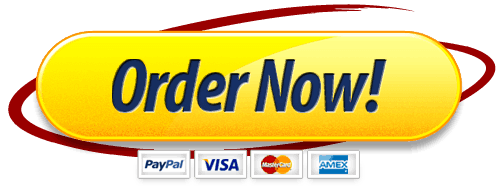