
Feasibility Assessment of Liquid Vancomycin Formulation
Purpose
The purpose of this project was to assess the feasibility of creating a liquid ready-to-use vancomycin product with a 10 – 12 month shelf life at 5⁰C. Multiple approaches were taken to determine appropriate excipient(s) for new formulation, including: NMR, HPLC, and computer modeling.
Background
Vancomycin is effective against gram-positive bacteria by binding to D-alanine-D-alanine residues in the bacterial cell wall, preventing cross-linking and promoting cell lysis. There is a current unmet need in hospital pharmacies to have liquid ready-to-use vancomycin premix. Not only will this ease pharmacy workflow but will more importantly give us the opportunity to produce higher dose vancomycin products (1.5 g in 350 mL and 2 g in 400 mL) which are currently unavailable.
Recently, a competitor has gained FDA approval for a liquid-stable vancomycin product, whose shelf life is 16-months when stored below 25⁰C in aluminum overpouch and 28-days at room temperature when removed from overpouch. A competitor sells this product in the following doses: 500 mg in 100 mL, 1 g in 200 mL, 1.5 g in 350 mL, and 2 g in 400 mL. However, due to its formulation, their product contains excipients known to cause embryo-fetal toxicity: N-Acetyl-D-Alanine (NADA) and polyethylene glycol (PEG) 400 (Table 1). Therefore, the Competitor’s product is a reproductive hazard to pregnant women. This black box warning gives us the opportunity to create a competitive liquid-stable vancomycin solution without hazardous excipients.
Figure 1. Vancomycin HCl (CAS 1404-90-6)
Table 1. Competitor’s Vancomycin Pre-mix Formulation
Compound | Amount in a 100 mL solution | Molar ratio | Comments |
Vancomycin HCl | 500 mg | 1 | Active Pharmaceutical Ingredient |
Polyethylene Glycol (PEG) 400 | 1.8 mL | 0.01 | Due to dose, warning of fetal malformations in animals |
N-Acetyl-D-Alanine (NADA) | 1.36 g | 30 | Known to cause fetal malformations in animals |
L-Lysine HCl | 1.26 g | 25 |
Approach 1: Nuclear Magnetic Resonance
Our current Vancomycin product shelf life is currently limited by the formation and precipitation of degradation products: DamS (des-amido-succinimido-Vancomycin B) and CDP1 (des-amido-isoaspartate-Vancomycin B) Major and minor (Figure 2). Our current Vancomycin Injection product shelf life is 12 months frozen and once thawed: 1 month at 5⁰C and 72 hours at room temperature.
Figure 2. Vancomycin undergoes an intramolecular reaction to form DamS and CDP1
Our goal was to find alternative excipients to stabilize vancomycin and prevent degradation. Using 13C NMR, our first approach was to calculate the binding affinities of various excipients to vancomycin. Excipients were chosen based on permutations around NADA, which is known to have a low dissociation constant from vancomycin based on literature (Ref 1).
For the NMR experiments, each excipient was tested at 5 concentrations or more while vancomycin concentration was kept constant at 5 mg/mL. pH was adjusted to 5.0 for all solutions to reflect final product target pH. Using a 850MHz NMR, 13C NMR spectra were generated for each solution with the help of one of our associates. The dissociation constant (Kd) for each excipient was calculated by measuring significant carbon peak shifts in the NMR spectra (Figure 3) and plotting the data on a Scathard Plot. If no chemical shifts in the carbon peaks were observed, Kd values could not be calculated and instead the excipient in question was determined to have no binding interactions with vancomycin. Table 2 below summarizes the calculated Kd values of all excipients tested.
Figure 3. 13C NMR spectra for vancomycin (bottom, black) and vancomycin + increasing concentrations of N-acetylglycine (increasing concentration from bottom to top).
Example of carbon peak shifts: original peak locations are denoted with red star while the blue star indicates the vancomycin carbon peak shift after ligand interaction.
Table 2. Summary of calculated Kd values at pH 5.0 with 5 mg/mL Vancomycin
Excipient Name | Kd (mM) at pH 5.0 | Comments/Concerns |
N-Acetyl-D-Alanine | 1.8 | Reproductive hazard |
N-acetyl-D-norvaline | 5 | |
N-acetyl-D-phenylalanine | 6 | Low solubility |
N-acetyl-glycine | 11.6 | |
N-(methylsulfonyl)-Alanine | 13.5 | Precipitation likely |
Sodium D-Lactate | 21.8 | |
Acetoxyacetic acid | 36.2 | |
Succinic acid | 36.9 | |
Sodium L-lactate | 45.2 | |
Levulinic acid | 71.4 | |
Sodium Acetate | 78.8 | |
Aspartic acid | 90.7 | |
N-acetyl-L-alanine | 95.3 | |
Glycine-glycine | 100.8 | |
D-Alanine | 103.7 | |
L-arginine | 109.8 | |
Gly-Asp | 111.9 | |
Glycine | 138.9 | |
N-Acetyl-Proline | 173.7 | |
L-histidine | 173.9 | |
L-lysine | 175.4 | |
Bicine | 254.3 | |
Tryptophan | no binding | proton NMR data suggests π-π stacking |
Asparagine | no binding | |
Threonine | no binding | |
Serine | no binding | |
N-acetyl-L-methionine | difference is too small to measure | |
N-acetyl-L-arginine | difference is too small to measure |
The carbon atoms with the most significant peak shifts in our NMR spectra correlate well with the reported bacterial cell wall binding sites (Figure 4). The calculated Kd values are in good agreement with literature values (Ref 1), confirming the high confidence in our method.
The NMR data set was forwarded to our collaborator at another runiversity to develop a model predicting excipient binding affinity to vancomycin based on the calculated Kd values. In parallel, this data set was used to identify high binding excipients to be tested with vancomycin under stressed conditions and analyzed via HPLC.
Figure 4. Vancomycin carbon atoms that experienced significant shifts upon binding to excipient relative to literature reported binding sites to bacterial cell wall.
Approach 2: HPLC
Based on the Kd values calculated via 13C NMR, excipients were selected to test their ability to stabilize vancomycin under stressed conditions. During the initial screen, vancomycin concentration was kept constant at 5 mg/mL (3.4 mM) and excipient concentration was kept constant at 100 mM. This 1:30 molar ratio of vancomycin to excipient was used to replicate our competitor’s formulation ratio of vancomycin to NADA. For simplicity, saline and dextrose were not added to the formulation during initial feasibility experiments. For all feasibility solutions, pH was adjusted to 5.0 with hydrochloric acid or sodium hydroxide before being flame sealed in 10 mL glass ampules.
Ampules were stored at 5⁰C or 40⁰C before being analyzed by HPLC according to 11-25-22-007 (Ref 2). The %peak areas for Vancomycin and the major degradants (DamS, CDP1 minor, and CDP1 major) are shown below in Figures 5 & 6. This data was combined from several ELN entries: 20191003-051, 20191010-044, 20191016-063, and 20191101-035. Two additional excipients: L-Tryptophan and N-acetyl-L-Tyrosine were also evaluated using a different analysis of the HPLC data; experimentation and results for L-Trp and N-acetyl-L-Tyr are further described in Appendix 1.
Figure 5. %Peak areas for Vancomycin control (black bar) or Vancomycin + excipient (blue bars) stored at 5⁰C for 5 days.
Figure 6. %Peak areas for Vancomycin control (black bar) or Vancomycin + excipient (blue bars) stored at 40⁰C for 5 days.
Several conclusions can be made from this data set. First, the excipients’ ability to stabilize vancomycin and prevent degradation correlates positively with the Kd values determined by NMR. Excipients that bind tighter to vancomycin and have a lower Kd value are better able to prevent degradant growth over time and across temperatures. This gives us confidence that the other institution generated computer model that is based on our NMR-generated Kd values should be able to accurately predict additional stabilizing excipients (see Approach 3 for more information). Second, similar trends in %peak areas are observed when comparing the 5⁰C and 40⁰C data; however, at high temperature, some lower binding excipients promote degradation compared to the vancomycin control, especially DamS. Although the exact reason for this is unclear, it is thought that since these excipients have higher Kd values, vancomycin could be held in a geometric position where the intramolecular DamS reaction is favorable when the excipient dissociates, which would happen more frequently. Since an increase in the rate of DamS formation would lead to a shorter shelf life than the current frozen product, these excipients were not analyzed further.
The two excipients whose stabilizing ability was most similar to NADA were N-acetyl-D-norvaline (NADN) and sodium D-lactate. Due to the structural similarity between NADA and NADN, there is concern that NADN could also potentially be a reproductive hazard. To determine its toxicity, five non-clinical studies would be required: Embryo-fetal development studies in rats and rabbits, ames genotoxicty, and 2-week repeat-dose toxicity studies in rats and dogs. Overall, the estimated cost for these non-clinical studies combined is $1.7 MM and would take ~9 months to complete. On the other hand, lactate is an FDA approved inactive ingredient and is also an ingredient in some of our products, such as Lactated Ringer’s solution (310 mg sodium lactate per 100 mL solution). Lactate as the vancomycin excipient would require minimal non-clinical studies, minimizing cost and time to development. While lactate shows promise, concentration of lactate should be a focus in formulation development. Lactic acidosis could be a concern for some patient populations if lactate concentrations are too high in our formulation. Conversations with clinical on appropriate excipient dosing should be maintained.
My next goal was to determine if D-Lactate was still effective in preventing vancomycin impurity growth at lower concentrations in addition to comparing the effects of D-lactate and racemic lactate (from lactic acid). For this experiment, vancomycin concentration was 5 mg/mL and pH adjusted to 5.0 for all formulations made. A 7-day incubation was performed at 5⁰C and 25⁰C using the following D-lactate or racemic lactic acid concentrations: 0, 10, 50, and 100 mM. Solutions were stored in flame sealed glass ampules during incubation and prepared and analyzed by HPLC (Ref 2; ELN 20191105-047).
Results from the 7-day incubation at 25⁰C are shown in Figure 7 (Data from 5⁰C incubation are in Appendix 2 Figure E). As depicted, the stabilizing effect of D-lactate and racemic lactate was relative to its concentration; higher lactate concentration resulted in reduced vancomycin degradation rates. While 100 mM D-Lactate had the greatest effect, lower concentrations were still able to significantly prevent impurity formation (except for DamS at 25⁰C). In addition, the data shows D-lactate to have a much greater effect at preventing degradation than racemic lactate, confirming the importance of a D-stereocenter. This makes sense since vancomycin has a high binding affinity for the bacterial cell wall D-Ala-D-Ala residues.
This data confirms that D-lactate is still a viable option at lower concentrations (30 mM or less) and is much more potent at lower concentrations than racemic lactate, especially at higher temperatures. Additional titration experiments are needed to confirm an exact D-lactate concentration.
I next wanted to determine if a combination of excipients would have an additive effect in preventing vancomycin degradation. Four excipients were chosen based on structural similarity to lactate: D-lactate, L-(+)-tartaric acid, DL-malic acid, and ethylenediaminetetraacetic acid (EDTA). Each excipient was either tested alone (at 30 mM) or in combination with one other excipient (at 15 mM each). Formulations were sealed in glass ampules and stored at 25⁰C for 3 or 8 days before being prepared for HPLC (Ref 2; ELN 20191209-090).
As shown in Figure 8, the two excipient formulations that were most successful in preventing vancomycin degradation were 30 mM D-lactate and 15 mM D-lactate + 15 mM EDTA. The three excipient formulations that did not prevent degradation when compared to the vancomycin control were 30 mM DL-malic acid, 30 mM L-(+)-tartaric acid, and 15 mM L-(+)-tartaric acid + 15 mM DL-malic acid. These findings helped eliminate the further development of tartaric acid and malic acid and instead shifted the focus to D-lactate and EDTA.
In parallel with the previous study, EDTA was further investigated as a stabilizing excipient at various concentrations: 1, 5, 10, 50, 100, and 200 mM (ELN 20191126-049). These various EDTA concentrations were incubated with 5 mg/mL vancomycin at 40⁰C for 6 days or 5⁰C and 25⁰C for 7 or 21 days before being run on HPLC (Ref 2). The resulting data for the 25⁰C incubation is shown in Figure 9; product limits are represented by dashed lines. Data from the 5⁰C and 40⁰C incubation temperatures are included in Appendix 2 Figures F & G.
Figure 7. %Peak areas for Vancomycin + D-lactate (blue bars) or Vancomycin + racemic lactate (grey bars) stored at 25⁰C for 7 days.
During the incubation, vancomycin degradation decreased as the EDTA concentration increased, with 200 mM EDTA being the most successful at preventing degradant formation. While the vancomycin and CDP1 %peak areas increased rapidly between day 7 and 21, DamS increased slowly, which is most likely due to a fast rate of conversion of DamS to the CDP1 degradants. In addition, EDTA appeared to stimulate DamS formation when compared to the vancomycin control in a concentration dependent manner: DamS concentration increased as EDTA concentration rose from 1 to 10 mM and then decreased from 10 to 200 mM EDTA. I suspect that a combination of lactate and EDTA will be necessary to obtain the longest shelf life for ready-to-use liquid vancomycin.
Figure 8. %Peak areas for Vancomycin ± excipient stored at 25⁰C for 3 or 8 days.
Vanco = vancomycin; D-Lac = D-lactate; TA = L-(+)-tartaric acid; MA = DL-malic acid; EDTA = ethylenediaminetetraacetic acid
Figure 9. %Peak areas for Vancomycin ± EDTA stored at 25⁰C for 7 or 21 days.
To determine the ideal ratio of D-Lactate to EDTA, a variety of concentrations were tested for both excipients (Table 3).
Table 3. Formulation components with 5 mg/mL Vancomycin at pH 5.0
Formulation | D-Lactate conc. (mM) | EDTA conc. (mM) |
1 | 0 | 0 |
2 | 10 | 20 |
3 | 15 | 15 |
4 | 20 | 10 |
5 | 25 | 5 |
6 | 30 | 0 |
7 | 30 | 10 |
8 | 30 | 30 |
9 | 30 | 60 |
Formulations were incubated for 3 and 21 days at room temperature in flam-sealed glass ampules before being prepared for HPLC (Ref 2; ELN 20191216-037). Results are shown in Figure 10; product limits are represented by dashed lines.
Figure 10. %Peak areas for Vancomycin ± D-lactate and EDTA stored at 25⁰C for 3 or 21 days.
As the ratio of D-lactate to EDTA increases, vancomycin degradation decreases. D-Lactate was chosen to have a maximum concentration of 30 mM in this formulation due to concerns of lactic acidosis in patients. As EDTA is added to the 30 mM D-lactate solution, there is a smaller but still notable effect on preventing vancomycin degradation. This data confirms the importance of lactate in the formulation, and that EDTA can provide further stability.
Approach 3: Modeling
We partnered with a researcher from the other institution to develop a computer model to predict excipient binding affinity to vancomycin based on a training set of Kd values provided by us. The reseacrher created five bi-parameter orthogonal models using a variety of chemical parameters (LogP, electronegativity, etc). Figure 11 compares the predicted Kd using a generated model and the calculated Kd from the training set.
Figure 11. Comparison of predicted Kd using di-parameter orthogonal model and measured Kd from NMR training set
Table 4. Comparison of the five bi-parameter models
Model | N | R2 | Q2 | Parameters |
1 | 20 | 0.88 | 0.81 | IFD-XPG; Es-Sum-CH3 |
2 | 20 | 0.89 | 0.82 | IFD-XPG, LogP |
3 | 20 | 0.89 | 0.80 | IFD-XPG, Es-Sum-ssNH |
4 | 20 | 0.90 | 0.84 | IFD-XPG, QM/MM -Elect |
5 | 20 | 0.87 | 0.82 | IFD-XPG, FISA |
In Table 4, all five bi-parameter models are compared. R2 and Q2 are two metrics used to evaluate the performance of a Partial Least Squares regression model. The R2 value is known as the “goodness of fit” and tells us how well we are able to mathematically reproduce the data from the training set using our model. The Q2 value is used validate the predictive ability of the model and is calculated in a similar way to R2 except using test data. All five models built by Dr. Mishra show an excellent predictive ability to determine the Kd of excipients, with a Q2 of 0.80 – 0.85.
With this high predictive ability, we propose completing a Phase II proposal with Northwestern University to computationally assess alternative vancomycin stabilizers. With this model, we can quickly determine binding ability of hundreds of ligands without the need to perform NMR or HPLC, saving time and money at the feasibility stage. Moving forward, we will focus our efforts on predicting the Kd of ligands that are already present in marketed products. For example, the next targets for Northwestern to analyze should include any remaining amino acids and derivatives that were not tested by NMR or HPLC.
Conclusions & Future Directions
There is an unmet need in hospital pharmacies to have ready-to-use liquid vancomycin solution. Currently, our frozen vancomycin product is not immediately accessible to patients, and our technology does not allow for bag volumes greater than 200 mL, limiting our dosing amount. The competitor has created a room temperature stable liquid vancomycin product, but uses excipients known to cause fetal malformations to achieve their long shelf life. Our goal was to find an alternative excipient that would result in a 5⁰C ready-to-use vancomycin solution with a shelf life of 10-12 months.
Using a combination of NMR and HPLC, we systematically explored a variety of excipients. I ultimately identified two excipients: D-lactate and EDTA that were shown to have a positive effect on preventing vancomycin degradation during short-term stressed conditions. Both excipients exist in marketed products and are considered inactive ingredients by FDA. One concern with using lactate as an excipient is the possibility of inducing lactic acidosis in patients, so concentration must be selected carefully. Both D-lactate and EDTA prevent vancomycin degradation in a dose-dependent manner, and it is therefore recommended to include one or both excipients at the maximum concentration clinical allows to obtain the longest possible shelf life.
The following are my recommendations for immediate next steps in this project. First, we must continue conversations with clinical and legal to determine what the maximum daily doses and maximum product concentrations would be for sodium D-lactate, sodium DL-lactate, and EDTA if used in a 400 mL 5 mg/mL vancomycin product. These excipients and concentrations must also be compared against any pre-existing patents to determine IP opportunities or FTO. In addition to those conversations, we must determine if a supplier of parenteral grade D-lactate is available. If not, we may be limited to racemic lactate (sodium DL-lactate), which is currently an ingredient in our Lactated Ringer’s solution and can be purchased parenteral grade. If we continue with a maximum lactate concentration of 30 mM, that would mean ~15 mM D-lactate and ~15 mM L-lactate. To obtain 30 mM D-lactate, double the amount of racemic lactate would have to be used (60 mM instead of 30 mM). If this is not possible, either due to patent conflicts of lactic acidosis concerns, then I would suggest maximizing [EDTA] as well as [sodium DL-lactate] to as high as clinical allows. It may also be beneficial to add PEG 400 (as seen in the our competitor’s formulation) to aide in solubility and prevent PM.
Once excipients and their concentrations have been confirmed with clinical and legal, we should immediately batch test formulations to make units for a 6-month stability study. Each formulation would be prepared in 5% dextrose solution and pH would be adjusted to 5.0 with HCl or NaOH. Osmolality should be confirmed to be within the nominal range (Alert limit: 272 – 308 mOsm/kg). Currently, the proposed formulations include one control and three test solutions (Figure 12).
Figure 12. Proposed formulations for 6-month stability study
Maximum concentration for D- or DL-lactate, EDTA, and PEG-400 would be determined in collaboration with our clinical and legal teams. In addition to these control and test formulations, replicating the Competitor’s formulation and putting up test units would be a good idea to compare against the competitor product. These batches would be filled into sterile plastic 50- or 100-mL containers. Units would be stored at either 5⁰C or 25⁰C. The following Tables 5 & 6 depict the anticipated unit numbers, time points, and test assays.
Table 5. Vancomycin Room-Temperature Storage (25oC) Test Intervals
(indicating number of replicate units of test and/or control articles)
Test Description | Method | 0-week | 1-week | 4-week |
Visual Inspection | Ref 3 | See
0-month in Table 6
|
3x test
3x control |
3x test
3x control |
pH | Ref 4 | 3x test
3x control |
3x test
3x control |
|
Chemical Purity | Ref 2 | 3x test
3x control |
3x test
3x control |
|
Particulate Matter | Ref 5 | — | 3x test |
Table 6. Vancomycin Refrigerated Storage (5oC) Test Intervals w/ Short Term
(indicating number of replicate units of test and/or control articles)
Test Description | Method | 0-month | 3-month | 3-month
w/ short term |
6-month | 6-month
w/ short term |
Visual Inspection | Ref 3 | 3x test
3x control |
3x test
3x control |
3x test
3x control |
3x test
3x control |
3x test
3x control |
pH | Ref 4 | 3x test
3x control |
3x test
3x control |
3x test
3x control |
3x test
3x control |
3x test
3x control |
Chemical Purity | Ref 2 | 3x test
3x control |
3x test
3x control |
3x test
3x control |
3x test
3x control |
3x test
3x control |
Particulate Matter | Ref 5 | 3x test | 3x test | 3x test | 3x test | 3x test |
Short Term = hold at 72 hours at 25⁰C
Upon completion of this study, we will have confirmed if our test formulations will be able to uphold a shelf life of 10 – 12 months at 5⁰C. Next steps would be to move this program forward into development.
References
- M. Pearce, U. Gerhard, and D. H. Williams. “Ligands which Bind Weakly to Vancomycin: Studies by 13C NMR Spectroscopy” J. Chem. Soc. Perkin Trans. 2 1995 p. 159-162.
- Test Method Assay 11-25-22-007. HPLC test method for the composition of vancomycin HCl drug substance & drug product and identification of vancomycin B in vancomycin injection drug product. Effective date: 24-Apr-2019.
- Test Method Assay D1-21-09-406, Visual Inspection of Samples from Development Studies, Current Issue.
- Test Method Assay 11-21-16-003, pH – Potentiometric Determination – USP, Current Issue.
- Test Method Assay 11-21-16-005, Particulate Matter Determination Using Light Obscuration Particle Counters, Current Issue.
ELN References to all Data
NMR Studies
20190814-103– NMR sample prep for the following excipients: acetate, D-Lactate, and D-Ala. Note: [Vanco] was not 5 mg/mL for this experiment. Copying Pearce paper conditions.
20190826-053 – NMR sample prep for the following excipients: N-acetyl-D-Ala, N-acetyl-Gly, and D-Ala
20190829-046 – NMR sample prep for the following excipients: acetate, D-lactate, and L-Trp
20190903-056 – NMR sample prep for the following excipients: N-methylsulfonyl-Ala, L-Asn, L-Asp, and N-acetyl-Pro
20190905-053 – NMR sample prep for the following excipients: L-His, L-Lys, L-Thr, and L-Ser
20190919-056– NMR sample prep for the following excipients: L-lactate, N-acetyl-D-Phe, N-acetyl-D-Norvaline, N-acetyl-L-Ala, and L-Arg
20190924-057– NMR sample prep for the following excipients: Gly-Gly, N-acetyl-D-Leu, N-acetyl-L-Met, and N-acetyl-L-Arg
Tryptophan Studies
20190805-046 – HPLC comparing vancomycin (5 mg/mL) ± 0.5% Trp filtrate vs concentrate after initial spin down in Pall Centrifugal Device
20190808-092 – HPLC comparing vancomycin (5 mg/mL) + Trp (0.0245 M or 5 mg/mL) or Ala (0.0245 M or 2.18 mg/mL). Comparing samples that were not filtered and samples that were washed for 60 min (x1 vs x2) with Pall Centrifugal Device
20190814-041 – HPLC comparing vancomycin (5 mg/mL) + 0.5% Trp samples that were not washed, washed and spun down for 30 min (x1 – x4), or washed and spun down for 60 min (x3) with Pall Centrifugal Device
20190828-087 – 2 day incubation at 65⁰C for vancomycin (5 or 10 mg/mL) ± Trp (1:1 and 5:1 molar ratio to vancomycin)
20190904-040 – HPLC run for samples prepared in 20190828-087. Samples were either taken “as is” or washed once using Pall Centrifugal Device before HPLC prep
20190905-097 – 1 day incubation at 65⁰C for vancomycin (0.5 mg/mL) ± Trp (1:1 and 5:1 molar ratio)
20190906-068 – HPLC for 20190905-097. Samples were washed x4 using Pall Centrifugal Device
20190916-055 – HPLC after 3 day 5⁰C or 40⁰C incubation of vancomycin (5 mg/mL) ± 0.5% Trp. Comparison of No wash or 30 min washes (x5 or x6) using Pall Centrifugal Device with reconstitution vol of 1 mL vs 2 mL.
20190923-038 – HPLC after 3 day 5⁰C or 40⁰C incubation of vancomycin (5 mg/mL) + 0.9% NaCl ± 1% Trp. No washes were performed prior to HPLC.
20190924-041 – HPLC after 2 day 5⁰C or 40⁰C incubation of vancomycin (5 mg/mL) + 0.9% NaCl ± 1.5% Trp. Comparison of No wash or 30 min washes (x6) using Pall Centrifugal Device.
20191001-057 – HPLC after 7 day 40⁰C incubation of vancomycin (5 mg/mL) ± 1% Trp or 100 mM D-Lactate. No washes were performed prior to HPLC.
Other Excipient Studies
20190820-039 – HPLC to determine if there is a RT shift when NADA binds to vancomycin (evaluated as an alternative to NMR binding studies)
20191003-051 – 5 day 5⁰C or 40⁰C incubation of vancomycin (5 mg/mL) with 100 mM N-acetyl-D-norvaline
20191009-104 – RTS#2 Sample Preparation for HPLC
20191010-044 – HPLC after 5 day 5⁰C or 40⁰C incubation of vancomycin (5 mg/mL) with 100 mM acetoxyacetic acid, succinic acid, levulinic acid, or N-acetyl-D-Ala
20191016-063 – HPLC after 5 day 5⁰C or 40⁰C incubation of vancomycin (5 mg/mL) with D-Lactate (10, 50, or 100 mM) or D-Lactate (100 mM) + 10 mM buffer (Citrate, Phosphate, or Acetate)
20191101-035 – HPLC after 5 day 5⁰C, 25⁰C, or 40⁰C incubation of vancomycin (5 mg/mL) with N-acetyl-L-Cys, N-acetyl-L-Tyr, or ethyl acetamidoacetate
20191105-047 – HPLC after incubation at 25⁰C for 7 days for vancomycin (5 mg/mL) + D-lactate or lactic acid (10, 50, or 100 mM)
20191113-072 – HPLC after incubation at 25⁰C for 7 days for vancomycin (5 mg/mL) + 100 mM L-(+)-tartaric acid, D-(-)-tartaric acid, DL-malic acid, D-(+)-malic acid, or EDTA
20191122-051 – HPLC after incubation at 5⁰C or 25⁰C for 3 and 10 days for vancomycin (5 mg/mL) + D-lactate, L-lactate, or DL-lactate (1, 2.5, 5, or 10 mM)
20191125-076 – RTS#2 Sample Preparation for HPLC
20191126-049 – HPLC after incubation at 5⁰C (7 & 21 days), 25⁰C (7 & 21 days), or 40⁰C (6 days) incubation of vancomycin (5 mg/mL) with EDTA titration (1, 5, 10, 50, 100, and 200 mM)
20191209-090 – HPLC after incubation at 25⁰C for 3 and 8 days for vancomycin (5 mg/mL) + 30 mM total combinations of D-Lactate, tartaric acid, malic acid, and/or EDTA.
20191216-037 – HPLC after incubation at 25⁰C for 3 and 21 days for vancomycin (5 mg/mL) + D-lactate & EDTA (various ratios).
20200102-030 – RTS#2 Sample Preparation for HPLC
Vanco “Lyo in a Bag” Class C data
20190715-088 – HPLC for Stability Study One T3 & Stability Study Two T1 samples
20190717-091 – pH for Stability Study One T3 & Stability Study Two T1 samples
20190918-088 – HPLC for Stability Study Two T2 samples
20191015-080 – HPLC for Stability Study One T4 samples
20191015-113 – pH for Stability Study One T4 samples
20191205-038 – HPLC for Stability Study Two T3 samples
Appendix 1 – Tryptophan & N-acetyl-L-Tyrosine
Tryptophan
L-Tryptophan (L-Trp) was identified as a potential excipient to prevent impurity formation in a ready-to-use liquid vancomycin product. My goal was to test the feasibility of L-Trp as an excipient in liquid vancomycin.
An initial concern emerged from our NMR data (see Approach 1 above). There were no measurable chemical shifts in the 13C NMR spectra, indicating no binding of L-Trp to vancomycin. To determine if there were any interactions outside of the vancomycin binding pocket, proton NMR was also run on the same L-Trp formulations. Peak broadening was observed in the aromatic region of the 1H NMR spectra between 6 – 8 ppm (Figure A). This suggests an interaction between the aromatic protons of vancomycin and tryptophan, such as π-π stacking; however, the binding constant could not be measured quantitatively.
Figure A. Proton NMR spectra of L-tryptophan (top), Vancomycin + L-tryptophan (middle), and vancomycin (bottom)
To determine if the aromatic interactions between vancomycin and L-Trp are enough to prevent degradation, HPLC was used to analyze short term incubation studies. However, a problem quickly became apparent in the HPLC chromatograms containing Trp. Using the validated HPLC method in SOP 11-25-22-007 (Ref 2), Trp has a retention time of ~5 min and due to its high concentration, the Trp peak covers several vancomycin impurity peaks, including degradant CDP1 minor (Figure B). This makes analysis via the current validated HPLC method impossible when Trp is present.
Figure B. HPLC chromatograms of Vancomycin (black) and Vancomycin + 1% L-Tryptophan (blue)
To overcome this obstacle, efforts were made to develop a pre-HPLC technique that would remove Trp from solution. The technique with the most promise was centrifugal filtration using a Pall Centrifugal Device with a 1k molecular weight cut off (MWCO) filter. Centrifugal devices are used to concentrate nucleic acid or protein samples and are typically used for buffer exchange or desalting samples. A 1k MWCO would allow Trp (MW 204) to easily pass through the filter while vancomycin (MW 1449) would be retained above the filter. Unfortunately, the MW of vancomycin is very close to the filter MWCO. Ideally, its recommended that a MWCO be selected that is three to six times smaller than the molecular weight of the solute being retained. Since a ≤ 500 MWCO centrifugal device was not available, some bleed through of vancomycin was expected when using the 1k MWCO. Another possible technique was to use dialysis, in which smaller MWCOs would be available. However, the long sample incubation times in the dialysis tubing (~24 hours) would not be practical in a manufacturing setting.
While initial results using the centrifugal device looked promising, there were several problems that would make using this technique less than ideal. The first issue is the overall length of time for sample preparation. To minimize the Trp peak in the HPLC chromatogram, I found that up to 6 washes with deionized water were necessary following the initial spin down. While I was able to reduce the time of each spin from 60 minutes to 30 minutes, sample preparation for HPLC still took approximately 5 hours to complete. Even following this time intensive sample preparation, a small Trp peak could still be observed in the HPLC chromatogram overlapping with the CDP1 minor peak. Also, as mentioned above, vancomycin does flow through the filter, though to a smaller degree than Trp. Unfortunately, each impurity flows through the filter at a different rate relative to vancomycin. We would have to calculate the rate of flow through for each impurity to be able to correctly compare the results following filtration and HPLC. Finally, I noticed that about 25% of the time, the filter rips during centrifugation, negating all efforts at purifying the sample.
Moving forward, to analyze Trp properly using %peak areas, I would suggest modifying the HPLC method to remove the Trp peak instead of trying to physically separate it pre-HPLC. Two variables that could be altered are mobile phase pH (shifting the mobile phase pH to be more basic) and column temperature (increasing the temperature). Note that if the HPLC method is changed, the method needs to be re-validated.
Before starting to alter the method, I first studied the effects of L-Trp (50 mM) vs D-lactate (100 mM) on vancomycin degradation using the current HPLC method. Samples were made with 5 mg/mL vancomycin and pH adjusted to 5.0. Tryptophan has low solubility at room temperature (1.14% or ~56 mM) and requires the solution to be heated to be fully dissolved before vancomycin is added. Sodium lactate is much more soluble at room temperature (~1300 mM) and therefore can be used at higher concentrations. 100 mM sodium D-lactate was used to replicate the competitor’s formulation ratio of vancomycin to NADA.
The samples were incubated at 5⁰C or 40⁰C in flame-sealed glass ampules before being prepared for HPLC without any centrifugal filtration (Ref 2; ELN 20191001-057). Instead of comparing %peak areas between formulations, total peak areas were compared for vancomycin, DamS, and CDP1 Major. CDP1 minor was not compared due to the overlapping Trp peak.
As shown in Figure C, L-Trp shows little/no improvement in retaining vancomycin and preventing impurity formation when compared with D-lactate. D-lactate as well as other excipients discussed in Approach 2 (EDTA, tartaric acid, etc) do not require any alterations to the current HPLC method; therefore, L-Trp was not pursued further as an excipient for vancomycin.
Figure C. 7-day incubation at 5⁰C (blue) or 40⁰C (orange) for vancomycin ± excipient
N-acetyl-L-Tyrosine
Another alternative excipient tested also had conflicting peaks visible in the HPLC chromatogram (N-acetyl-L-tyrosine). Similar to L-tryptophan, total peak area for vancomycin and CDP1 Major were compared following a 5-day incubation at 5⁰C, 25⁰C, or 40⁰C (Figure D) (ELN 20191101-035).
Figure D. 5-day incubation at 5⁰C (greys), 25⁰C (greens), or 40⁰C (blues) for vancomycin ± excipient
Unfortunately, neither N-acetyl-L-Cys nor N-acetyl-L-Tyr prevent vancomycin degradation (top graph) and have mixed results depending on temperature for CDP1 Major (bottom graph). Similar to L-Trp, these excipients did not show enough promise to continue with modifications to the HPLC method.
Appendix 2: Additional Data
Figure E. %Peak areas for Vancomycin + D-lactate (blue bars) or Vancomycin + racemic lactate (grey bars) stored at 5⁰C for 7 days.
Figure F. %Peak Area for Vancomycin ± EDTA stored at 5⁰C for 7 or 21 days.
Figure G. %Peak areas for Vancomycin ± EDTA (various conc.) stored at 40⁰C for 6 days.
What Students Are Saying About Us
.......... Customer ID: 12*** | Rating: ⭐⭐⭐⭐⭐"Honestly, I was afraid to send my paper to you, but you proved you are a trustworthy service. My essay was done in less than a day, and I received a brilliant piece. I didn’t even believe it was my essay at first 🙂 Great job, thank you!"
.......... Customer ID: 11***| Rating: ⭐⭐⭐⭐⭐
"This company is the best there is. They saved me so many times, I cannot even keep count. Now I recommend it to all my friends, and none of them have complained about it. The writers here are excellent."
"Order a custom Paper on Similar Assignment at essayfount.com! No Plagiarism! Enjoy 20% Discount!"
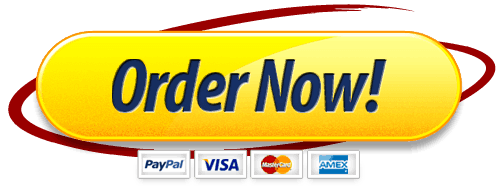